Information Theory and Creationism
Spetner and Biological Information
By Ian Musgrave
Posted August 17, 2003
Introduction
Dr. Lee Spetner is an information theorist who has written a book
claiming that random mutations can not produce the kind of "informational"
changes in biology that is allegedly required by evolution (1). It is
interesting that in a book supposedly about information theory, the classic
formulation of information theory of Shannon and Weaver (2) does
not get mentioned. Spetner's notion of information in biology has been taken up
by several groups of evolution deniers, and while others have produced specific
critiques of his work (3,4), there
is no overall general analysis of his arguments.
In this review I will consider if Spetner's metrics can be validly applied to
biology, and how Spetner actually applies them to real world examples. Although
his arguments are superficially plausible, a closer look with some knowledge of
biochemistry shows significant flaws. I will first briefly describe Spetner's
metric of information, I will then show that 1) Spetner's metrics depend on a
binding mechanism that does not occur in nature, 2) that Spetner's metrics
require that substances bind to enzymes in an all or nothing fashion, whereas
real substrates do not bind in this way. Furthermore, I will show that Spetner
himself is inconsistent in his application of his metrics. In his Xylitol
example he does not actually use the measure he develops, and in the streptomycin
example he swaps to a different metric, when his original metric would show
increased information. Finally, I will show that his "directed evolution" model
is based on a misunderstanding of one form of random mutation.
Glossary
active site: | a ligand
binding site (usually a fold or cleft in the structure of an enzyme) where
chemical reactions take place. | |
amino acid: | a small organic
molecule used as a building block for peptides or proteins. | angstrom: |
A very small distance; 10-10 meter. | enzyme: | a catalytic protein that performs chemical
modification of a substrate
that binds to its active
site. | ligand: | any molecule that binds
to a specific binding site on an enzyme or receptor. | peptide: | an organic molecule made up of chains of
amino acids. Proteins are long peptides. | protein: | a large organic molecule made up of
chains of amino acids. | receptor: | a
protein that binds ligands
which are hormones or neurotransmitters. Binding of the hormone results in
activation of an enzymatic pathway, but the hormone itself is not
chemically modified (unlike a substrate). | substrate: | a ligand
molecule that binds to an enzyme's
active
site and is chemically modified by the enzyme. |
Overview of Spetner's
Metrics
Classical information theories such as Shannon's Information theory has been
applied to biology by several authors. Those that have addressed evolution have
concluded that evolution can, under appropriate circumstances, increase
"information" in Shannon's sense (eg see 13). Dr.
Spetner however claims that random mutations cannot increase "information". In
his claim, Spetner uses two separate metrics of information. One is an
"expectation" measure whereby an ensemble of different strings has less
information that an ensemble of identical strings. This will surprise people
familiar with standard Shannon Weaver
information or Algorithmic
information, but is a valid formulation under particular circumstances. I
will not go into this in any depth because Spetner himself doesn't.
He also uses an "addressing" measure of information. This is fairly simple to
understand. "Brisbane" has less information than "Cannon Hill, Brisbane"
which has less information than "Richmond Road, Cannon Hill, Brisbane" which
has less information than "666, Richmond Road, Cannon Hill, Brisbane"
There is a formal way to do this using binary addresses for matrix elements
in n x n (or n x n x n) matrices. It's a bit of a stretch mapping this onto
substrate binding, but there is nothing inherently more loony about this than
mapping telegraphy transmission to DNA replication. A key feature of this
measure is that "information" is directly related to string length. That is, a
longer string has more information in both the "word enzyme" (see below) and
binary addressing formulations. Another important feature is that binding is
"all or nothing". I discuss the implications of this in detail in Spetner's
word-enzyme example.
Enzyme-substrate
binding and Spetner's specificity
Enzymes are protein catalysts that promote chemical reactions on the
compounds that bind to them (their substrates). As enzymes bind strongly only to
a very few chemicals out of the multitudes present in their environment, they
are considered to be highly specific. Spetner claims that enzyme specificity is
analogous to addressing specificity, and that enzymes with many substrates have
less information than enzymes with one substrate. In his explanation uses a
"word enzyme" thought experiment rather than a real example, and we will examine
this argument in detail later.
However, at first glance it seems like a reasonable argument. Enzyme-substrate
(or hormone-receptor) binding is likened to a "lock and key" mechanism, where
the substrate is the key and the enzyme is the lock. (important caveat, both the
lock and key are "floppy" as enzymes and their substrates are somewhat
flexible).
Figure 1: Binding of ligands to proteins like enzymes or
hormone receptors has been likened to a "lock and key" arrangement, where the
molecule fits into a space in the protein in a similar manner to a key fitting
into a lock. While a useful analogy to help us visualise things, this image
can be misleading as we will see, as various binding interactions are
important, not just physical "lumps and bumps".
Spetner's binding
specificity is not only a property of enzymes
Note that Spetner's argument is a general one, and applies not only to
enzymes, but to any specific binding system, such as hormone/receptor
interactions, binding between cytoskeletal elements etc, regardless of whether
the substrate is acted on enzymaticaly. In the following discussion I will be
using the term "ligand" which covers everything that binds specifically to an
acceptor site, which includes substrates.
Note further that there is a subtle shift in the argument. One can easily see
that the address for "666, Richmond Road, Cannon Hill, Brisbane" takes more
information to specify than "Brisbane", but the claim that enzymes have more
information because they bind only one substrate is similar to the claim that
"666, Richmond Road, Cannon Hill, Brisbane" has more information than "Brisbane"
because fewer letters are delivered there than there are to "Brisbane". This is
a somewhat different claim, that the information of the physical object at an
address has the information required to specify that address.
Spetner's
requirements are not found in real world systems
But accepting Spetner's claim, it might seem intuitively obvious that a more
specific lock accepting only one key has more information a less specific lock
which accepts many keys. This is actually incorrect, but rather than go into
this here see this
paper on cryptography and master keys: WARNING 5Mb file. For the
moment we will accept this though. Analogously we might expect an enzyme is more
specific because it has more binding points than an enzyme that is less specific
(and there is also the unstated assumption that single substrate enzymes are
more important than multi substrate enzymes. They are not).
For example we might imagine an active site that uses 4 binding points has
more information than one with two binding points
Skip text
graphic
X--O O--Y X--0 O--Y
\ / \ /
DRUG DRUG
/ \
Z--N N--Y
Where X, Y and Z are amino acids in the enzymes active site.
Unfortunately, biology doesn't work that way. The number of binding points
are important, to a degree. But other physical properties of the enzyme are
important too. For example, take the betalactamase enzymes that break down the
antibiotic cephalosporins. One mutant variant can breakdown extended
cephalosporins (where the molecule has been made more bulky), where as the
normal enzyme can't. The mutant variant doesn't have fewer binding points than
the normal enzyme, but has a more flexible hinge so that the catalytic group can
reach the lactam ring easier.
Worse still, you can have substrates which bind at exactly the same number of
points, but which bind more firmly because you have substituted a chlorine atom
for an oxygen atom, and changed the distribution of electrons on the drug
molecule.
Binding specificity is not something simply analogous to "addressing", it
involves physical shape (which is amenable to an addressing analysis) and
physicochemical properties (which is not simply amenable to an addressing
analysis, especially when the properties are those of the substrate) and
structural properties of the enzyme, like hinge flexibility or helix distortion
which are also not amenable to an addressing analysis (does a helix which flexes
to the right have more information than a helix which flexes to the left?)
Simply put, any analysis of the "information" of a protein that addresses the
substrate binding specificity, although superficially plausible, is naive in the
extreme, because substrate specificity is not amenable to the proposed analysis
(especially when some of that information is in the substrate). Also, his
analysis deals solely with single substrate enzymes, how he deals with
bi-bi-random two substrate two product enzymes I don't know, he seems to feel
that all enzymes should be like (some of) the enzymes of the Krebs citric acids
cycle.
Having outlined in general why Spetner's metric is not applicable to
protein-ligand binding, in the next section I will examine Spetner's word enzyme
example in depth, and compare this to a well studied receptor-ligand system, the
angiotensin II receptor.
Application to real
biological systems:
Spetner's word-enzyme
example
Lets examine this in some detail and revisit his "word enzyme" example. This
differs in key ways from the binary addressing example he gives first, but I'll
ignore that for this article, as the "word enzyme" is the example he uses to
bridge the gap between real systems [Streptomycin
in his example] and the binary addressing measure, see (1) pages
134-137.
Spetner's Information is
proportional to binding string length
Here is Spetner's word enzyme "ghts". This can "bind" many "substrates"
(actually ligands, as this is a general binding argument): ghts
Nights
Lights
Fights
Rights
(and many more, omitted for clarity)
Lightship
Nightshade
by increasing the string length, we reduce the number of "ligands" "bound",
increasing "specificity" ghtsh
Lightship
Nightshade
by increasing the string length again, we reduce again the number of
"ligands" "bound", increasing "specificity" yet again. ghtshi
Lightship
At each stage, for every "increase in specificity", the bit length of the
binding string increases. Thus the "information" in the binding string
increases.
Note that although Spetner has claimed that random mutations cannot produce
increases in information, in his "word enzyme" example random mutations can
increase information. Simply randomly adding a letter to the string "ghts" will
result in a number of strings that bind substantially fewer "ligands". It will
also result in a number of stings that bind no ligands, but it is enough to note
that simple random mutation will produced "word enzymes" of higher
"specificity"
However, when we come to real systems, the link between increased binding
"string" length and "specificity" just doesn't hold. An example I have given
above, a change in a hinge region, far from the binding site, can allow in
bigger ligands, even though the actual binding sequence is unchanged. Skip text graphic
a--d--------| a--d-------| a--d--------|
abcd n VS abcd N abcdE N
bc----------| bc---------| bc----------|
In the
section that follows I will examine this in greater depth.
Spetner's Information
depends on "all or nothing" binding
As noted above, Spetner's information is proportional to number of
substrates, but Spetner never defines substrate. This is not trivial. As we have
seen above Spetner's "enzyme" either binds a ligand, or it doesn't. This is
important as the whole nexus of Spetner's argument is that the number of
substrates reflects the length of the binding string, and the length of the
binding string, in bits, is the information of the enzyme/receptor/binding
protein. However,in the real world the binding of a ligand to a protein is
not an all or nothing affair, substrates have varying degrees of
"stickyness", which is not addressed by Spetner's metric. Even a very specific
enzyme will bind one ligand very tightly, a few not so tightly, and a great
number very weakly. One cannot simply say that only very strongly bound ligands
will be considered, as very weak ligands may be of great physiological
importance, if they are present in a high enough concentration (there are many
examples of this in physiology, in the angiotensin II receptor example below,
there are a group of peptides called the histidine triad peptides, which though
being very weak binders of the angiotensin II receptor, do indeed modulate the
receptor activity under physiological circumstances as their concentrations are
so high in the body). This has a theoretical and a practical implication.
Theoretically, this means that there is no necessary connection between
string length and the number of ligands bound, as "stickyness" depends on
molecular factors that do not easily translate into information. Does a string
containing aspartic acid have more or less information than one containing
asparagine, when virtually the only difference between them is charge?
"Stickyness" also depends on some molecular features that are part of the
substrate. Practically, it means that Spetner information cannot be measured
experimentally, since we can never truly know the total number of substrates
unless we test all potential substrates in the universe.
Thus we can see that Spetner's metrics have are unlikely to be able to
measure the information content of enzymes/receptors/binding proteins at all. In
the next section I make an in depth analysis that drives this point home.
Word-enzymes compared
to real proteins
Let's take as an example the important hormone receptors, the angiotensin II
(AT) receptors. The AT receptors come in two versions, coded by separate genes,
the AT1 receptor is protein made of a chains of a 359 amino acids, and the AT2
receptor is a 363 amino acid long protein. Both bind the peptide angiotensin II
(AII) and related peptides, but AII binding to the receptors stimulates entirely
different enzyme systems (AII binding to the AT1 receptor activates the enzyme
phospholipase C and the binding to AT2 activates a different enzyme,
phosphatase). Despite almost identical binding profiles, the receptors have only
34% of the amino acids in their structure in common. We have a very good
understanding of the structure and properties of the AII binding site in the
angiotensin receptors, so they provide a platform for testing Spetner's ideas
about specificity.
AII and similar ligands bind to the same amino-acid sequence in the AT1 and
AT2 receptor. We can use the one letter amino acid code, where a single letter
substitutes for an amino acid name, (eg D is the amino acid aspartate, see
below), to represent the binding sequence as a string, analogous to Spetner's
word binding string ghtshi. The angiotensin receptor binding string is
DNKH. So these receptors look like a good example to test Spetner's ideas. The
amino acids in the binding string are not contiguous, but are separated by many
amino acids (5, 6).
The amino acids that make up the sequence DNKH:
D(Aspartate 281) N(Asparagine 111) K(Lysine 199) H(Histidine 256)
AT1 D(Aspartate 297) N(Asparagine 126) K(Lysine 215) H(Histidine 273) AT2
Note that the amino acid's are in different positions in the different
receptors (eg , Aspartate 281 is at position 281 in the 359 amino-acid long
chain that is the AT1 receptor and Aspartate 297 is at position 297 in the 363
amino-acid long chain that is the AT2 receptor) partly because the AT2 receptor
has a longer C-terminus, and also due to an insertion into the sequence between
N and K and between K and H in the AT2 receptor. Thus the concept of "precise
sequence" is not exactly applicable to receptor binding. The amino acids are
bought close (but not into a linear sequence, more like a ring) by the three
dimensional folding of the proteins, and this three dimensional folding is
bought about by very different sequences.
Figure 2: How proteins fold to give three dimensional
structures that bring amino acids in proximity. Left hand panel,
schematic showing how the helicies brings the DNKH sequence together (only 4
helicies shown for illustration purposes). Right hand panel, the three
dimensional cartoon structure of the AT1-receptor with AII bound. We are
looking at the receptor from above, with some chains removed for clarity. The
transmembrane helicies are shown as grey coils, AII is shown in yellow, the
DNKH amino acids are shown in red. See http://home.mira.net/~reynella/chime/ang_tuta.htm for 3D
structures (needs MDL CHIME
plug-in to view and manipulate the 3D structure).
Skip
text diagram
H H---H H--|
ED EN EK EH |
L L L L |
I I I I |
X X X---X |
|--------------|
This is problematic for Spetner's claims, as the sequence DNKH depends on a
number of factors that are NOT dependent on that sequence, thus the "true"
information content is not reflected by the binding sequence alone. Again note
that the three dimensional folding is not rigid, and flips between a number of
different states (Protein chains are somewhat flexible, more like a ball of
semi-cooked spaghetti than the rigid shapes they are sometimes drawn as, thus
enzymes and receptors are "floppy" locks and ligands are "floppy" keys: this is
relevant to Spetner's claims, more later).
As I said above, the AT receptors bind angiotensin and similar peptides to
the ligand binding site. As with the receptor, not all of the ligand sequence
binds to the binding site. The sequences are shown in the one letter amino acid
code, with the amino acids that bind being shown in bold. AII DRVYIHPF
AIII RVYIHPF
SarIle SRVYIHPI
SarAsp SDVYIHPI
CGP NYKRHPI
AIV VYIHPF
For AII and AIII, the peptides bind to the receptor binding site sequence
thusly (5,6,7,8):
RYF DNKH
Broadly analogous to ghtshi-lightship "binding". F actually binds to both K
and H. This is mostly electrostatic interactions rather than the physical "bumps
and hollows" Spetner uses in his (incorrect) analogy for streptomycin binding.
The basic amino acid R binds electrostatically to the acidic amino acid D, Y and
N form hydrogen bonds and F forms a pi bond with H, and a hydrogen bond with Y.
(In SarAsp, the acidic D interacts with the acidic D, but dipole charge
distribution means that they bind rather than repel. Chemistry and common sense
do NOT go well together. (5,6)
But as we look at SarIle and SarAsp, we can see Spetner's model of binding is
going badly wrong. These have only two of the three matching points present in
AII and AIII, yet they bind very well indeed. Maybe only the DN binding sequence
is critical. AIV, which lacks the D binding region, still binds (not as well as
the others, but it still binds, more on this later (5,6,7,8).
In terms of Spetner's model, it is as if "ghtshi" bound Lights, Nights, and
Ship as well as lightship. This breaks the nexus Spetner has tried to form
between the binding string length and number of ligands bound.
Figure 3: Binding of ligands to the AT1 receptor, looking down
on the receptor from the top. Circles represent transmembrane helicies (see
figure 2). Some transmembrane helicies have been omitted for clarity. Top
panel, Binding of Angiotensin II to the AT1 receptor, the N terminal
region of angiotensin is represented by R for simplicity. The D(Asp 281) N(Asn
111) K(Lys 199) H(His 256) binding sequence is shown in RED (as for figure 2),
other amino acids that are peripherally related in stabilizing the molecule
are shown in BLACK. Bottom panel: Binding of the ligand losartan to the
AT1 receptor. The common binding sequence between AII and losartan is shown in
BLUE, other amino acids that are peripherally related in stabilizing the
molecule are shown in BLACK. Note that different amino acids are
involved in losartan binding.
Worse yet, CGP binds to completely different amino acid's in the ligand
binding cleft, so there is a further disconnect from Spetner's model, as there
is more than one "binding string" in a ligand binding site. This is not an
unusual phenomenon, and is also true of many receptors. Figure 2 shows the
comparison between the binding points of AII and losartan in the AT1 receptor,
showing the differences.
Numbers of ligands
bound is not simply related to binding string length
So, what happens when we shorten the binding string DNKH. According to
Spetner's model, you should increase the number of ligands bound. We can mutate
the amino acid H to A (alanine), Q (glutamine) or R (arginine). The Q mutation
keeps charge and size, but loses the pi bonding, the R mutation keeps the
charge, the A mutation loses charge and size, thus mutations from H to R and A
are like truncating the binding string to DNK. In the AT1 receptor, the binding
doesn't change with any of these mutations. When we mutate H to Q or R in the
AT2 receptor, all binding is lost (7,8).
Either way, Spetner's model is dead wrong about what happens when the "string
length" of a ligand binding string is shortened (and this again invalidates his
measure).
Even worse, what happens when you mutate N in DNKH (Asparagine at position
111 in the AT1 receptor and position 126 in the AT2 receptor)? If you mutate N
to G (glycine) a very short neutral amino acid, unlike the longer charged
asparagine, there is no contact with the ligand and the G, so the binding
sitestring becomes DKH. Despite this truncation, you still bind AII, AIII, etc.,
but AIV has become "stickier"(something which Spetner doesn't actually address
in his metric). As I mentioned before, the AT receptors flop between several
conformational shapes. The N->G mutation restricts the receptor to fewer
conformational shapes, making it MORE specific. It turns out that AII and
AIII bind to all the conformational shapes that the enzyme flops between, but
AIV binds only to one of the forms, that stabilised by the N111G mutation (5,6). Thus
binding is a critical function of 3D shape, not covered by Spetner's metric, and
a single mutation can increase specificity (as according to Spetner's criteria,
a protein that has one conformational shape has more specificity than one with
multiple conformational shapes).
What if we take Spetner's metric at face value, looking ONLY at the
numbers of ligands bound. Are there other examples of mutations that can
increase "specificity" in the AT receptors. Yes. In the AT2 receptor, replacing
the amino acid Y (tyrosine) at position 215 in the AT2 chain with R wipes out
CGP binding, leaving the AII and SarIle binding intact (note that Y is
NOT part of the DNKH binding string). Conversely, mutation of Y215 to Q
wipes out AII and SarIle binding, while leaving the CGP binding intact (here
"wipes out" means that there is no significant binding when 1 mM of liagand is
present). Thus we can see that single mutations can increase "specificity", as
in the number of ligands bound. Thus, even with Spetner's invalid metric,
we can still show that random mutation increases "specificity".
We have seen that in real world examples, Spetner's requirements for
substrate number to be a indicator of protein information are not met, and
Spetner's metric is invalid. We will now turn to the examples Spetner presented
in his book, and show how his own analyses of these examples fail.
Spetner's examples
Lets now look at how Spetner himself applies his metric.
Xylitol metabolism
Given the extensive discussion Spetner gives to binding specificity, and his
thought experiments emphasising that specificity is equivalent to the number of
substrates bound, it may come as some surprise that in Spetner's analysis of the
ribulose to xylitol mutation he doesn't actually consider binding
specificity.
He does consider a biochemical measure called specificity, but this is
not Spetner's specificity measure. This is a ratio between catalytic efficiency
and binding specificity. Okay, you say now, this is pretty trivial, we can work
with this. Well, no. Catalytic efficiency is something else again. It is
definitely not amenable to an "addressing" measure, and is based on a range of
physicochemical properties such as the charge distribution in the
substrate, the free rotation of the catalytic side group (does a
catalytic group that rotates 10 angstroms have more or less information than one
that rotates 15 angstroms?) and so on. Critically, two substrates can have the
same binding specificity, but different catalytic efficacy which
confounds the information analysis.
Worse again, Spetner's argument is related to the number of substrates acted
on (see above). Yet in the ribulose dehydrogenase mutating to xylitol
dehydrogenase, in both cases the enzyme bound 3 substrates, by his own
definition no information change has occurred. The rate of
dehydrogenation has changed, but the number of substrates acted on does not
change. Two points need to be emphasised:
a) As noted in the previous section, Spetner's metric is a measure that says
an enzyme that binds substrate A and substrate B has less information than an
enzyme that binds substrate A alone (remember ghtsh,
which "binds" Lightship and Nightshade has less information than ghtshi which
only binds Lightship, because ghtshi is a longer string). This does NOT work
when we are comparing an enzyme that binds 20 molecules of substrate A for every
80 molecules of substrate B, with and enzyme that binds 80 molecules of
substrate A for every 20 molecules of substrate B. The length of the binding
string need not have changed, or changed in the direction that Spetner demands,
so the bit length measure is no help at all.
b) The relative magnitude of the change may be entirely due to changes in
catalytic efficiency, which has no information content in Spetner's addressing
scheme (nor can I see any meaningful way to calculate the "information" in this
with any existing information metric).
It gets worse still. Spetner compared the "specificities" of 3 substrates,
because they were the only substrates measured in the experiment. In reality
ribulose dehydrogenase (and xylitol dehydrogenase) binds a lot more substrates
than just those 3 (although the catalytic efficiency is very low for the
majority of substrates bound). As pointed out above, without assessing the full
panel of substrates, any claim about specificity is meaningless. (But which
substrates? if we restrict ourselves to natural substrates, we exclude xylitol,
a synthetic sugar not found in the natural environment, but developing xylitol
dehydrogenase activity was the whole point of the exercise) if we include
synthetic substrates, we have a potentially infinite number of substrates to
test. How do we know what is really happening?
It continues to get worse. Spetner assumed that the point reached in the
experiment (where Ribulose and Xylitol were being broken down at roughly similar
rates by the mutant enzyme), was as good as it gets, no further improvement was
possible. In the experiment he looked at, the main point was to see if xylitol
activity could be developed, not getting the optimum activity. In fact, in other
experiments mutant enzymes were produced that broke down xylitol 20 times faster
than ribulose (which kind of destroys his thesis see 9).
Thus the ribulose example does not support Spetner's thesis. Furthermore, we
have literally hundreds of enzymes where random mutation results in high
substrate specificity (there has been enormous amounts of work on developing
novel and specific enzyme activities from generalist alpha-beta barrel proteins
e.g. see 10, and
recently the evolution of specific binding activities from random peptides has
been reported 11).
Streptomycin
binding:
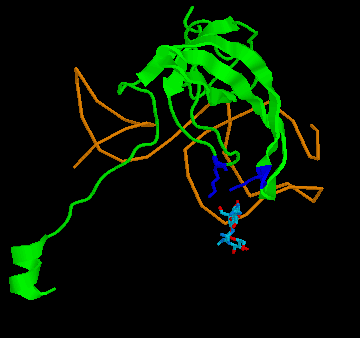
Figure 4: Binding of streptomycin to the S12 subunit of the
30S ribsomal subunit. Top panel, The S12 chain is shown in cartoon
format (green), with the rRNA shown as the backbone only (brown). The residues
critical for directly binding streptomycin, Lysine 42 and Lysine 87, are shown
in blue. Streptomycin is shown with carbon as grey, nitrogen as blue and
oxygen as red. Binding to the RNA is not shown for simplicity. Bottom
panel: the effect of the mutation on S12 structure. The replacement of
lysine 42 (yellow, on the white chain) causes the chain to twist away from the
streptomycin (pink chain), preventing binding.
His other example is the case of resistance to the antibiotic streptomycin.
Streptomycin kills bacteria by interfering with protein assembly on the
ribosome. Mutation of the rspL gene which codes for the S12 subunit of
the 30S ribosomal particle in bacteria can result in resistance to streptomycin.
The 30S ribosomal particle is a multi-subunit structure which in turn forms part
of the protein synthesizing ribosomal particle. The S12 subunit together with
the 16S RNA forms part of the proof reading center of the transfer RNA (tRNA)
acceptor binding site. Mutation of streptomycin-binding lysine at position 42 in
the protein chain that makes up the S12 subunit to threonine or asparagine
results in streptomycin failing to bind to S12, with resulting resistance of the
bacteria to the antibiotic streptomycin. This is a classic example of a
beneficial mutation, as is found in many textbooks as it was work on this
mutation that determined that mutations were random.
Antibiotic-resistant mutant ribosomes
have increased Spetner information
In terms of Spetner's binding metric, the mutant S12 protein is more
specific, it now only binds tRNA rather than tRNA and streptomycin. Now,
streptomycin isn't a substrate, so you might object to using binding specificity
in this case. However, Streptomycin binding is Spetner's own example for
binding specificity, and he uses it as an example of a "lock and key" binding
system (see above). Recall also that his metric is generally applicable to all
ligand-acceptor interactions (remember that in his "word enzyme" example, there
is just binding going on, so by his own examples the ligand doesn't have to be a
substrate).
Furthermore, the mutant does have an effect on the substrate-binding
accuracy of the ribosome. While streptomycin binds at at different site from the
actual tRNA proof reading site, this is a classic example of something called
allosteric modulation. In Spetner's simple model of substrate binding, only
ligands that directly bind to the active site are considered. However, many
enzymes and hormone receptors have sites for ligands distinct from the active
site, where binding of ligands to these distant sites results in modification of
substrate/ligand binding at the active site. These kinds of ligands are called
allosteric modulators, and are very important in biology. They include sodium
binding to a pocket on protein-digesting enzyme trypsin, far from the active
site, which alters trypsins catalytic activity and glycine binding to an
modulatory site far from the glutamate binding site on the glutamate receptor
(which alters nerve activity) and many other examples. If you exclude a lock and
key binding allosteric modulator of enzyme action like streptomycin from
consideration, then you are ignoring biology.
Streptomycin-binding ribosomes turn out garbage proteins because streptomycin
messes up the proof-reading centre (which is how streptomycin kills bacteria).
The mutant version which doesn't bind streptomycin is actually MORE
accurate, i.e. more SPECIFIC, than the wild type. The wild-type proof
reading centre makes a few mistakes even in the absence of streptomycin, and the
mutant forms make even fewer mistakes than the wild type( roughly 85% fewer; 12).
This is a clear increase in Spetner's binding specificity:
- The mutant gene product doesn't bind streptomycin at all (it has one
ligand rather than two)
- It binds the substrate peptidyl tRNA more accurately
- It catalyses more accurate peptide synthesis.
Does Spetner acknowledge this? No, Spetner now swaps to the
expectation measure and claims (without evidence) that since there must be more
S12 sequences that don't bind streptomycin than those that do, information must
have decreased (why didn't he do this analysis on the ribulose enzyme?).
However, he is dead wrong. The ensemble of all rsPL genes that produce
streptomycin binding S12 is around 1060. The ensemble of all
rsPL genes that don't bind streptomycin is around 1060
too (from an analysis of neutral mutations using the method of Yockey (13). So
the information difference is so small as to be non existent. If we only take
amino acid changes in the streptomycin binding amino acids, then there are
roughly the same number of substitutions that will allow binding as those that
don't (10 resistant versus 6 normal see 14).
Importantly, there is a particular mutation, AAA42 -> AGA42 (lysine to
arginine) which doesn't bind streptomycin AND has wild type accuracy and
translation rates(15).
This is the only possible (single) mutation that does this, so it's ensemble is
smaller than the wild type ensemble. Another example is the mutation that
results in the S12 subunit still binding streptomycin and being
resistant to streptomycin. Again, this is the only possible mutation, so by the
expectation measure this mutation has more information than the wild type.
Thus we see, that however Spetner applies his metrics, the
Streptomycin-resistant mutation has more information that the wild type
gene.
Spetner and "directed"
mutations:
Spetner believes he has shown that random mutations can not produce the
increases in information that would be needed in evolution (as we have seen, he
is wrong). Spetner proposes that evolution is via "directed" mutations (with the
implication that Divine Intelligence is in some way behind this "direction").
The apparent existence of "directed" mutations was based on some early work by
Barry Hall. However, Spetner did not follow up the more recent research on this
work, and misunderstands the origin and significance of "directed"
mutations.
Most people know that mutations arise at random in dividing cells, and that
the mutations occur at various places in the replication cycle as a consequence
of damage (e.g. from mutagenic chemicals or radiation) or proof reading errors
in the copying process.
However, most people are unaware that there is significant DNA turnover in
non-growing (non-dividing) cells (16).
Adaptive (directed) mutation are mutations that apparently result in the
selective appearance of favorable mutations. The designation of these mutations
has caused considerable controversy and they have been called adaptive,
directed, Cairnsian, selection-induced, and stressful lifestyle associated
mutations (SLAM). One researcher coined the name "Fred" while trying to find a
name that would not inflame the critics, and "Fred" seems to have found it's way
into at least informal discourse by the relevant researchers (17).
"Fred" occurs only under non-lethal selection in non-dividing cells, and has
been suggested to be a neo-Lamarkian mechanism for getting environmental
information into the genes. However, "Fred" is no such thing (16,17,18),
and is not directed in the sense that Spetner suggests.
- Fred is not Lamarckian. There is no reverse transcriptase involved,
mutants are not back transcribed from some environmentally altered protein or
even from a fortuitously modified mRNA.
- Fred is not directed, mutations are found randomly throughout the genome,
not just in the "adaptive" gene.
- Fred is dependent on recombination, damaging either of the recombination
enzymes RecA or RecBCD reduces the rate of "adaptive" mutations.
- Fred is dependent on DNA polymerase, in DNA polIII mutants with better
proof reading, the rate of "adaptive" mutations is reduced.
- Fred is largely depended on defective mismatch repair (MMR). Defects in
MMR increase "adaptive" mutations and increased MMR or over expression of MMR
reduces "adaptive" mutation. Crucially, MMR is reduced in non-growing and
stressed cells.
So the following model shows how "adaptive" mutations occur. Nutritionally
deprived non-growing cells are under stress, stress leads to double stranded
breaks in the DNA, recombination vis RecBCD primes the DNA synthesis, DNA PolIII
finishes the job but makes mistakes, which slip through because starvation has
largely turned off mismatch repair. This results in genome wide mutation at a
faster rate than normal, and the occasional mutant that can utilize the
"selective" substrate (16, 17, 18).
The canonical example is E. coli that have a crippled Lac gene,
and cannot utilize lactose, when plated on a medium that has only lactose as a
carbon source, the cells cannot grow, but after a time colonies appear that can
use lactose. These colonies contain a version of the crippled gene that has been
restored to function (usually by a 1 bp frameshift (16, 17, 18).
So Fred, while certainly exciting from the genetic point of view, turns out
just another boring random mutation.
Conclusion:
So to summarize, although Spetner's arguments are superficially plausible, a
deeper look with some knowledge of biochemistry shows massive flaws. Spetner is
wrong in the details of the biology, ligand specificity is not directly governed
by binding string length as required by Spetner's theory, and ligand binding is
not an "all or nothing affair". This invalidates his analyses. Even then,
Spetner's own examples do not support his claims. Furthermore, when using his
metrics Spetner swaps metrics when one shows inconvenient changes.
References:
1. Spetner, L. M. (1998) NOT BY CHANCE! Shattering the Modern Theory of Evolution,
Judaica Press, New York.
2. Shannon CE (1948) A Mathematical Theory of Communication. Bell Sys Techl J
27.
3. Schneider TD, (2000) Evolution of Biological Information, Nucleic
Acids Res, 28(14): 2794-2799.
4. Max E (2001) The Evolution of
Improved Fitness
5. Hunyady et al., (2003) Agonist induction and
conformational selection during activation of a G-protein-coupled receptor. [ PubMed] TIPS 24, 81-86
6. Le MT et al., (2002) Angiotensin
IV Is a Potent Agonist for Constitutive Active Human AT1 Receptors. J Biol
Chem, 277, 23107-23110
7. Knowle D, et al., (2001) Role of Asp297 of the AT2
receptor in high-affinity binding to different peptide ligands. [ PubMed] Peptides, 22, 2145-2149
8. Turner, CA, et al., (1999) Role of the His273 Located
in the Sixth Transmembrane Domain of the Angiotensin II Receptor Subtype AT2 in
Ligand Receptor Interaction. [ PubMed] BBRC, 257, 704-707
9. Hartley, B.S. (1984) Experimental evolution of
ribitol dehydrogenase. In R.P. Mortlock (ed.), "Microorganisms as Model Systems for Studying Evolution" (pp.23
- 54) Plenum, New York.
10. Matsumura I, Ellington AD. In vitro evolution of
beta-glucuronidase into a beta-galactosidase proceeds through non-specific
intermediates. [ PubMed] J Mol Biol. 2001 Jan 12;305(2):331-9
11. Hayashi Y, et al., Can an arbitrary sequence
evolve towards acquiring a biological function? [ PubMed] J Mol Evol. 2003 Feb;56(2):162-8.
12. Björkman J, et al., Novel ribosomal mutations affecting translational accuracy,
antibiotic resistance and virulence of Salmonella typhimurium. Mol
Microbiol. 1999 Jan;31(1):53-8.
13. Yockey HP. (1992) Information Theory and Molecular Biology, Cambridge University
Press. Chapter 6.3
14. Tolvonen JM et al., Mol. Micro. 1999,
1735-1746
15. Björkman J et al., (1998) Virulence of
antibiotic resistant Salmonella typhimurium. Proc Natl Acad Sci USA. 95,
3949-3953.
16. Foster PL & Rosche WA. Mechanisms of mutation
in nondividing cells. Insights from the study of adaptive mutation in
Escherichia coli [ PubMed] Ann N Y Acad Sci 1999, 870, 133-45.
17. Rosenberg, SM. Mutation for Survival [ PubMed] Curr Opinion Gen Dev 1997, 7:829-834
18. Rosche WA, and Foster PL. (1999 Jun 8). The role of
transient hypermutators in adaptive mutation in Escherichia coli. Proc Natl
Acad Sci U S A , 96, 6862-7.
Acknowledgments:
Many thanks to Chris Ho-Stuart, Michael Hopkins, Bill Hudson and Douglas
Theobald for helpful suggestions and proof reading.
Originally posted at Talk Origins.
|